Home>Science>The Surprising Speed At Which Planes Take Off And Accelerate On The Runway
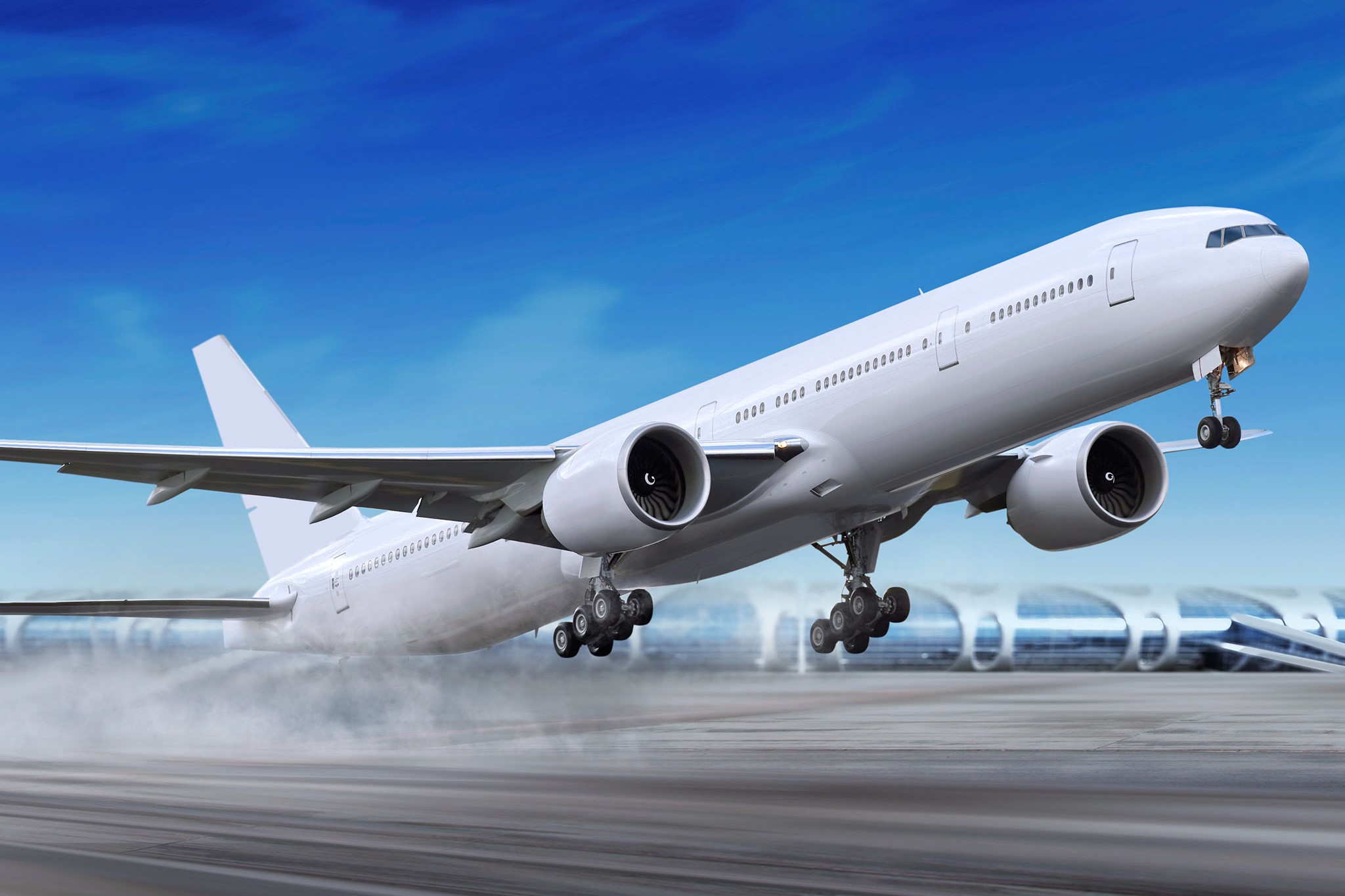
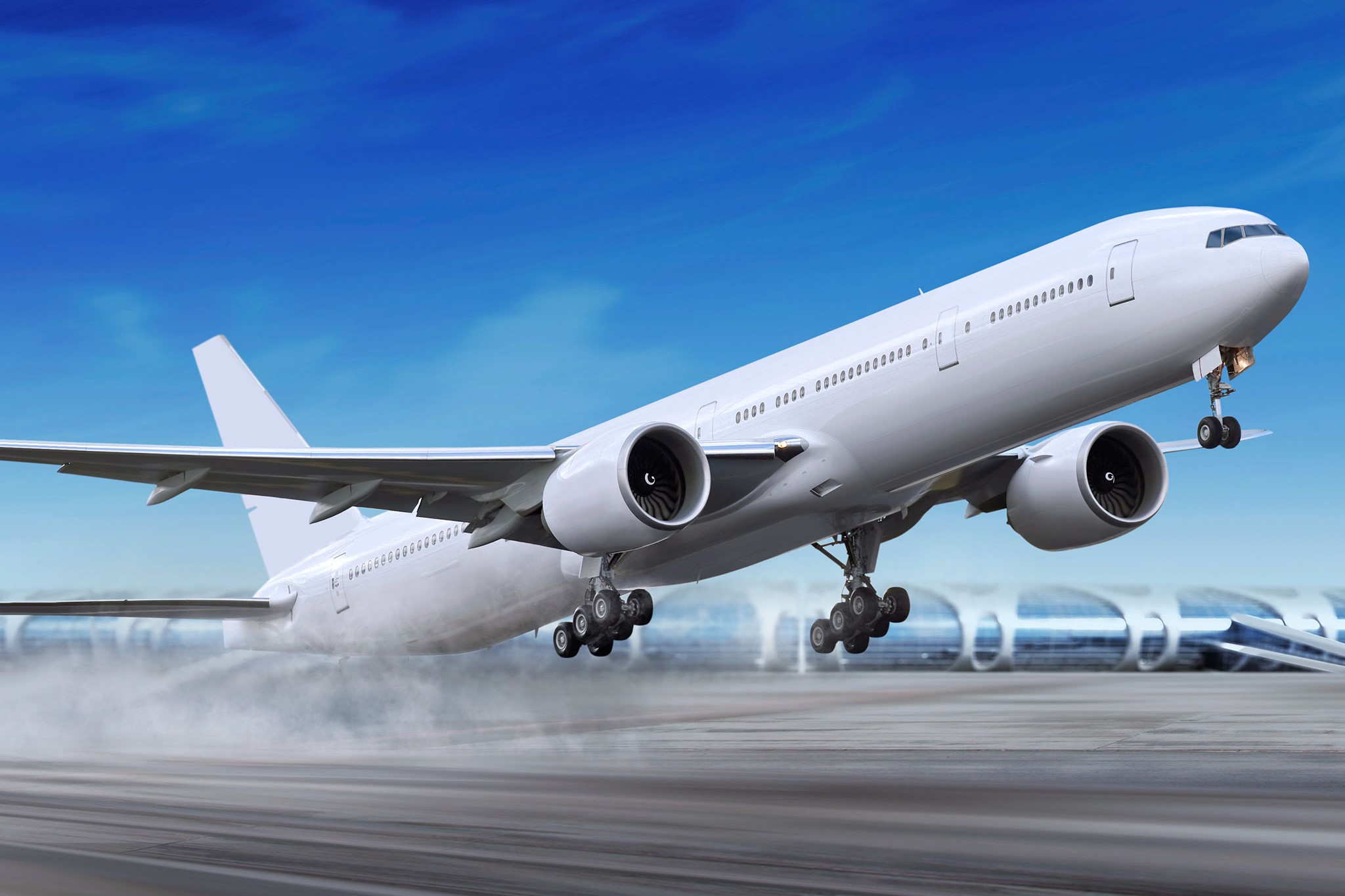
Science
The Surprising Speed At Which Planes Take Off And Accelerate On The Runway
Published: January 19, 2024
Discover the science behind the astonishing speed at which planes take off and accelerate on the runway. Explore the fascinating physics of flight in this insightful article.
(Many of the links in this article redirect to a specific reviewed product. Your purchase of these products through affiliate links helps to generate commission for Noodls.com, at no extra cost. Learn more)
Table of Contents
Introduction
Have you ever marveled at the incredible speed at which airplanes take off and accelerate on the runway? It's a captivating sight to witness a massive aircraft swiftly gaining momentum and lifting off into the sky. The physics behind this seemingly effortless feat is a fascinating blend of engineering, aerodynamics, and environmental factors.
As we delve into the intricacies of airplane takeoff and acceleration, we'll uncover the remarkable interplay of various elements that contribute to this impressive display of aviation technology. From the powerful thrust generated by jet engines to the influence of weight, air density, and runway length, each component plays a crucial role in determining the speed and efficiency of an aircraft's takeoff.
Buckle up as we embark on a journey to unravel the secrets behind the surprising speed at which planes take off and accelerate on the runway. Get ready to gain a deeper understanding of the forces at play and the remarkable engineering feats that enable these metal birds to defy gravity and soar into the boundless expanse of the sky.
Read more: Laid Off Vs Layed Off: Which Is Correct?
Factors Affecting Takeoff Speed
The takeoff speed of an aircraft is influenced by a myriad of factors, each playing a crucial role in determining the velocity required for a successful liftoff. Understanding these factors is essential for pilots, engineers, and aviation enthusiasts alike, as they shed light on the intricate dynamics of flight operations.
1. Aircraft Design and Configuration
The design and configuration of an aircraft significantly impact its takeoff speed. Variables such as wing shape, size, and the presence of high-lift devices like flaps and slats directly affect the lift generated during takeoff. Additionally, the placement and thrust capabilities of the engines contribute to the overall aerodynamic performance, influencing the speed at which the aircraft can achieve sufficient lift.
2. Environmental Conditions
Weather and environmental factors play a pivotal role in determining takeoff speed. Air density, which is influenced by altitude, temperature, and humidity, affects the aerodynamic forces acting on the aircraft. At higher altitudes or in hot and humid conditions, the reduced air density necessitates higher takeoff speeds to generate the required lift.
3. Weight and Payload
The weight of the aircraft, including fuel, cargo, and passengers, directly impacts the takeoff speed. Heavier loads require more lift, necessitating a higher velocity for the aircraft to become airborne. Pilots meticulously calculate the aircraft's weight and balance to ensure that the takeoff speed is within safe limits for the given configuration.
4. Runway Length
The length of the runway available for takeoff plays a critical role in determining the required speed for liftoff. Longer runways provide ample distance for acceleration, allowing aircraft to reach the necessary speed for takeoff more gradually. In contrast, shorter runways necessitate higher takeoff speeds to ensure a safe and timely departure.
5. Safety Margins and Regulatory Requirements
Aviation regulations and safety standards dictate minimum takeoff speeds to ensure the safe operation of aircraft. These requirements consider factors such as engine failure scenarios, obstacle clearance, and the need for sufficient margin in the event of unforeseen circumstances. Pilots adhere to these specified speeds to maintain a high level of safety during takeoff.
In essence, the takeoff speed of an aircraft is a meticulously calculated parameter that takes into account numerous variables, ranging from the aircraft's design and weight to environmental conditions and regulatory considerations. This intricate interplay of factors underscores the complexity of aviation operations and the meticulous planning involved in ensuring safe and efficient takeoffs.
The Role of Thrust in Acceleration
Thrust, the force generated by an aircraft's engines, plays a pivotal role in the acceleration phase of the takeoff process. As the aircraft taxis along the runway, the powerful jet engines unleash a torrent of thrust, propelling the aircraft forward and initiating the acceleration required for liftoff. This force is the driving factor behind the aircraft's ability to overcome inertia and gain the necessary velocity for takeoff.
Jet engines, whether turbofan or turbojet, are designed to intake air, compress it, add fuel for combustion, and then expel the hot, high-velocity exhaust gases to produce thrust. This process exemplifies Newton's third law of motion, where for every action, there is an equal and opposite reaction. In the context of aircraft propulsion, the expulsion of high-velocity gases from the engines results in a reactive force that propels the aircraft forward.
The magnitude of thrust generated by an aircraft's engines is carefully calibrated to ensure that it exceeds the total drag acting on the aircraft during takeoff. Drag, the aerodynamic resistance encountered by the aircraft as it moves through the air, encompasses various forms such as parasitic drag, induced drag, and form drag. By surpassing the drag forces, the engines enable the aircraft to accelerate, ultimately reaching the critical speed for takeoff.
During the acceleration phase, pilots meticulously monitor the thrust output to ensure that it aligns with the predetermined acceleration profile. This involves a delicate balance between engine power, airspeed, and the aircraft's weight, all of which influence the acceleration characteristics. The goal is to achieve a smooth and controlled acceleration, allowing the aircraft to swiftly reach the designated takeoff speed without excessive strain on the engines or airframe.
Furthermore, the role of thrust extends beyond the initial acceleration, encompassing the continuous propulsion required throughout the climb phase after liftoff. As the aircraft ascends, the engines sustain the necessary thrust to counteract the gravitational pull and propel the aircraft to its designated cruising altitude. This sustained propulsion underscores the enduring significance of thrust throughout the entire takeoff and ascent sequence.
In essence, the role of thrust in acceleration is indispensable, serving as the propulsive force that enables aircraft to overcome inertia, achieve the required takeoff speed, and ascend into the skies. The harmonious orchestration of engine power, aerodynamic forces, and pilot expertise culminates in the seamless acceleration of aircraft along the runway, setting the stage for the awe-inspiring moment when they gracefully take flight.
Impact of Weight on Takeoff Performance
The weight of an aircraft exerts a profound influence on its takeoff performance, shaping the dynamics of acceleration and lift during the critical phase of departure. As an aircraft prepares for takeoff, its weight, encompassing the combined mass of fuel, cargo, passengers, and the aircraft itself, directly impacts the speed required to achieve liftoff. This fundamental relationship between weight and takeoff performance is intricately woven into the fabric of aviation operations, dictating meticulous calculations and operational considerations.
Heavier aircraft necessitate a higher takeoff speed to generate the requisite lift for ascension. The added mass imposes greater gravitational force, compelling the aircraft to overcome a more substantial inertia during the acceleration phase. As a result, pilots and flight engineers meticulously calculate the takeoff speed, factoring in the aircraft's weight and the prevailing environmental conditions to ensure a safe and efficient departure.
Moreover, the distribution of weight across the aircraft, including the positioning of cargo and passengers, plays a pivotal role in maintaining the aircraft's balance and stability during takeoff. A well-balanced load distribution is essential for achieving optimal aerodynamic performance and mitigating the risk of potential imbalances that could compromise the aircraft's maneuverability.
In the realm of commercial aviation, the concept of maximum takeoff weight (MTOW) holds paramount significance. This defined limit represents the maximum allowable weight at which an aircraft can safely take off, factoring in considerations such as runway length, environmental conditions, and engine performance. Adhering to the prescribed MTOW ensures that the aircraft operates within safe parameters, mitigating the risks associated with excessive weight during takeoff.
Furthermore, the impact of weight on takeoff performance extends beyond the realm of commercial aviation to encompass military aircraft, where considerations of payload, weaponry, and mission requirements intricately intertwine with the dynamics of takeoff. The ability to swiftly and efficiently achieve liftoff, even under the constraints of substantial weight, underscores the remarkable engineering feats and operational prowess inherent in military aviation.
In essence, the impact of weight on takeoff performance permeates the core of aviation operations, shaping the intricacies of acceleration, lift, and operational safety. The meticulous management of weight considerations, coupled with the harmonization of engineering precision and operational expertise, underscores the enduring significance of this fundamental factor in the realm of aviation.
Read more: How To Take Oven Door Off
Influence of Air Density and Temperature
The influence of air density and temperature on aircraft takeoff performance is a fundamental aspect of aviation operations, intricately intertwined with the aerodynamic forces that govern the dynamics of flight. Air density, which refers to the mass of air molecules within a given volume of space, directly impacts an aircraft's ability to generate lift during takeoff. In essence, the density of the surrounding air significantly influences the aerodynamic forces acting on the aircraft, thereby shaping the speed required for a successful liftoff.
At higher altitudes, where air density is lower due to reduced atmospheric pressure, aircraft encounter a distinct set of challenges during takeoff. The diminished air density necessitates higher takeoff speeds to achieve the requisite lift, as the reduced mass of air molecules exerts a diminished force on the aircraft's wings. Pilots and flight engineers meticulously account for these altitude-related variations in air density, factoring them into the takeoff calculations to ensure that the aircraft can attain the necessary velocity for liftoff.
Temperature further compounds the intricacies of air density, as variations in thermal conditions directly influence the density of the surrounding air. Warmer temperatures result in decreased air density, posing additional considerations for aircraft takeoff performance. In hot and humid climates, the reduced air density necessitates higher takeoff speeds to compensate for the diminished aerodynamic forces acting on the aircraft's wings. This interplay between temperature and air density underscores the dynamic nature of aviation operations, requiring pilots and engineers to adapt their strategies to account for the environmental variables at play.
The influence of air density and temperature extends beyond the realm of commercial aviation to encompass diverse aircraft categories, including military and general aviation. Whether navigating through high-altitude terrains or contending with temperature differentials across various geographic regions, pilots and flight crews remain cognizant of the nuanced effects of air density and temperature on takeoff performance. This heightened awareness underscores the meticulous planning and operational acumen that define the realm of aviation, where the interplay of environmental variables and aerodynamic principles shapes the intricacies of flight operations.
In essence, the influence of air density and temperature on aircraft takeoff performance underscores the profound impact of environmental factors on the dynamics of flight. The harmonization of aerodynamic principles, altitude considerations, and thermal effects culminates in a comprehensive understanding of the interwoven forces that govern the speed and efficiency of aircraft takeoff, exemplifying the intricate fusion of science and engineering within the realm of aviation.
The Importance of Runway Length
The length of the runway available for aircraft takeoff and landing holds profound significance in the realm of aviation, exerting a pivotal influence on the speed, performance, and safety of flight operations. A runway's dimensions directly impact an aircraft's ability to accelerate, achieve liftoff, and land safely, making it a critical factor in the meticulous planning and execution of flight maneuvers.
In the context of takeoff, the importance of runway length becomes evident in its role as a determining factor for the acceleration distance required by aircraft to reach liftoff speed. Longer runways provide ample space for gradual acceleration, allowing aircraft to attain the necessary velocity for liftoff without imposing undue strain on the engines or compromising safety margins. This extended distance facilitates a smoother and more controlled takeoff, enhancing operational flexibility and mitigating the constraints associated with limited runway lengths.
Furthermore, the availability of sufficient runway length directly correlates with an aircraft's maximum takeoff weight (MTOW) capabilities. In the realm of commercial aviation, where aircraft are laden with passengers, cargo, and fuel, the prescribed MTOW necessitates corresponding runway length requirements to ensure safe and efficient takeoff operations. Adequate runway length enables aircraft to accommodate heavier loads, thereby expanding the scope of operational capabilities and enhancing the efficiency of flight operations.
Additionally, runway length considerations extend beyond the realm of standard takeoff scenarios to encompass critical contingencies such as rejected takeoffs and emergency procedures. In the event of an aborted takeoff, ample runway length provides pilots with the necessary distance to safely decelerate and bring the aircraft to a halt, mitigating the risks associated with high-speed abort situations. Similarly, during emergency landings, extended runway length offers pilots a wider margin for maneuvering and deceleration, bolstering the safety and resilience of flight operations.
The significance of runway length transcends commercial aviation to encompass diverse aircraft categories, including military, cargo, and general aviation. Whether facilitating the operations of large commercial airliners or accommodating the specialized requirements of military aircraft, runways serve as the foundational infrastructure that underpins the dynamics of flight. The harmonization of runway length with operational demands, safety considerations, and environmental factors epitomizes the meticulous orchestration of aviation infrastructure to support the seamless and secure conduct of flight operations.
In essence, the importance of runway length permeates the core of aviation operations, shaping the dynamics of takeoff, landing, and critical flight maneuvers. The strategic allocation of runway resources, coupled with meticulous planning and operational foresight, exemplifies the enduring significance of runway length as a cornerstone of aviation infrastructure, underscoring its indispensable role in enabling the safe, efficient, and resilient conduct of flight operations.
Conclusion
In conclusion, the surprising speed at which planes take off and accelerate on the runway is a captivating testament to the intricate interplay of engineering, aerodynamics, and environmental factors within the realm of aviation. From the critical role of thrust in propelling aircraft forward to the profound influence of weight, air density, temperature, and runway length on takeoff performance, the dynamics of flight operations unfold as a harmonious symphony of scientific principles and engineering precision.
The culmination of these factors underscores the meticulous planning and operational acumen that define the realm of aviation, where the interplay of environmental variables and aerodynamic principles shapes the intricacies of flight. Whether navigating through high-altitude terrains, contending with temperature differentials, or accommodating diverse aircraft categories, the profound impact of environmental factors on the dynamics of flight remains at the forefront of aviation operations.
Furthermore, the enduring significance of runway length as a cornerstone of aviation infrastructure exemplifies its indispensable role in enabling the safe, efficient, and resilient conduct of flight operations. The strategic allocation of runway resources, coupled with meticulous planning and operational foresight, underscores the pivotal role of runway length in supporting the seamless and secure conduct of flight operations.
Ultimately, the surprising speed at which planes take off and accelerate on the runway serves as a poignant reminder of the remarkable fusion of science and engineering within the realm of aviation. It embodies the relentless pursuit of innovation, precision, and safety that characterizes the aviation industry, perpetuating the awe-inspiring spectacle of flight and the enduring spirit of human ingenuity.